Stormwater Best Management Practices in an Ultra-Urban Setting: Selection and Monitoring
Fact Sheet - Dry and Wet Vegetated Swales
Traditionally, swale designs were simple drainage and grassed channels (Figure 17) that primarily served to transport stormwater runoff away from roadways and rights-of-way and provided inconsistent water quality treatment (Claytor and Schueler, 1996). Today, designers emphasizing water quality management are shifting from the drainage/grassed channel design concepts to carefully engineered dry/wet vegetated swale designs (Figure 17). Two general types of grassed swales are discussed in detail here-a dry swale, which provides water quality benefits by facilitating stormwater infiltration, and a wet swale, which uses residence time and natural growth to treat stormwater prior to discharge to a downstream surface water body.
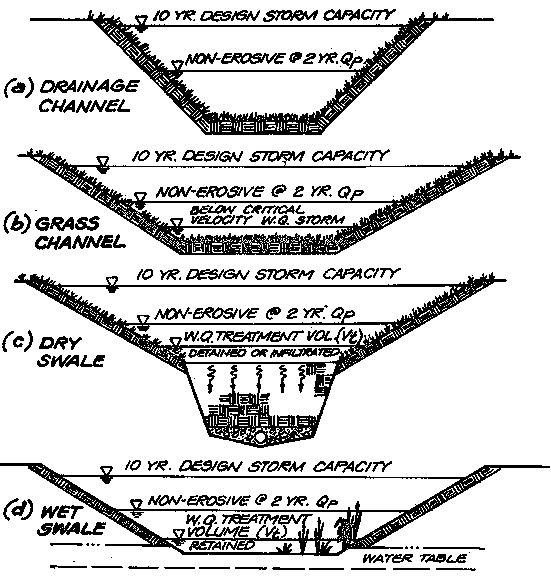
Dry swales are distinguished from a simple drainage/grassed channel by the addition of carefully selected, highly permeable soil (usually sandy loam), check dams, and an underdrain system (Figure 18). These design features ensure that infiltration of stormwater will not depend only on the infiltration rate of the existing natural soils. Only in special circumstances where natural soil and groundwater conditions consistently provide high infiltration will a traditional drainage/grassed channel design provide the same water quality benefits as a dry swale design.
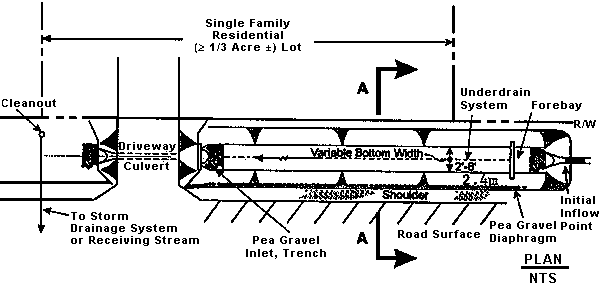
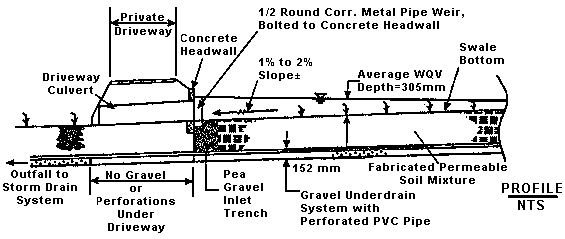
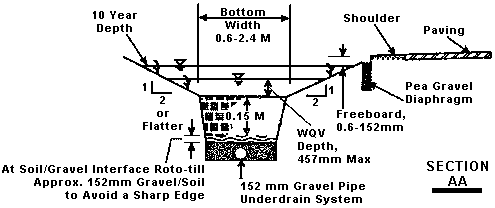
Wet swales are distinguished from the simple drainage/grassed channel by design features that maintain a saturated condition in soils at the bottom of the swale (Figure 19). The goal of a wet swale is to create an elongated wetland treatment system that treats stormwater through physical and biological action. Unlike dry swales, infiltration of stormwater is an undesirable condition in a wet swale because it would likely result in conditions detrimental to maintaining saturated soils to support wetland vegetation.
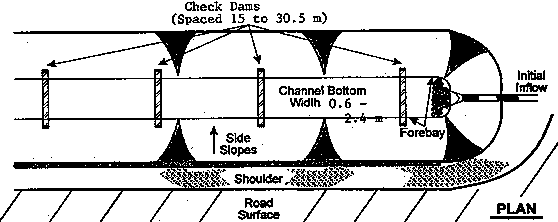
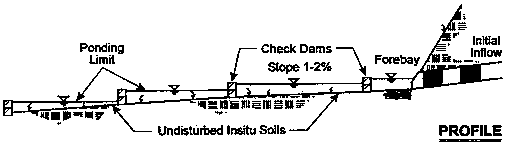
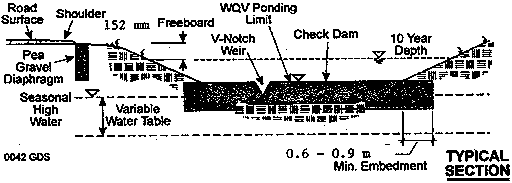
Applicability
Dry and wet swales are appropriate for use in narrow areas along roads and medians where sufficient space exists to accommodate the additional storage depth and width. These swales are relatively inexpensive BMPs, and the total cost is principally related to earth moving construction costs. Because drainage/grassed channels are commonly installed in roadway right-of-way areas to provide essential drainage, implementing a more complex dry or wet swale design usually results in a relatively small additional cost and provides significantly better water quality management. Where sufficient space is available in ultra-urban areas, either dry or wet swales may be appropriate BMPs.
The design requirements of swales are relatively flexible; the gradient, size, and shape are typically based on local regulations that ensure adequate conveyance of the stormwater. In most applications, swales are placed parallel to roadways and care must be taken to ensure they do not impose an unacceptable safety hazard to any vehicles that might leave the roadway. Swales are practically vandal-proof and add an aesthetic value to roadside areas as long as they are maintained and litter and debris are regularly removed. However, wet swales can create ideal breeding habitat areas for nuisance insects such as mosquitoes.
Effectiveness
Both dry and wet swales demonstrate good pollutant removal, with dry swales providing significantly better performance for metals and nitrate. Dry swales typically remove 65 percent of total phosphorus (TP), 50 percent of total nitrogen (TN), and between 80 and 90 percent of metals. Wet swale removal rates are closer to 20 percent of TP, 40 percent of TN, and between 40 and 70 percent of metals. The total suspended solids (TSS) removal for both swale types is typically between 80 and 90 percent. In addition, both swale designs should effectively remove petroleum hydrocarbons based on the performance reported for grass channels. See Table 15 for additional removal effectiveness rates for swales. Seasonal differences in dry/wet swale performance have been reported; pollutant removal efficiencies for many constituents can be markedly different during the growing and dormant periods (Driscoll and Mangarella, 1990). In seasonal climates, fall and winter temperatures force vegetation into dormancy, thereby reducing uptake of runoff pollutants and removing an important mechanism for flow rate reduction. Furthermore, decomposition of accumulated organic matter can lead to production of nutrients in a soluble form, making them free to be transported downstream. Freezing temperatures greatly reduce infiltration in dry swales, removing an important pollutant removal mechanism.
Table 15. Pollutant removal effectiveness for swales (%)
Study |
TSS |
TP |
TN |
NO3 |
Metals |
Comments |
City of Austin (1995)1 |
68 |
43 |
23 |
-2 |
|
Grassed channel |
Yu et al. (1993)2 |
21-95 |
32-85 |
- |
- |
- |
Vegetated swale |
Yu et al. (1994)2 |
49 |
33 |
- |
- |
13 |
Length of swale evaluated reduced to 100 ft |
Yu and Kaighn (1995)1 |
30 |
negligible |
- |
- |
11 |
Grassed swale |
Yousef et al. (1985)1 |
- |
(-48)-48 |
(-14)-25 |
- |
(-25)-92 |
Grassed swale |
Kahn et al. (1992)2 |
83 |
29 |
- |
- |
30 - 72 |
200 foot swale |
1Removal efficiencies based on concentrations.
2Removal efficiencies based on mass loading. |
There are limited data currently available on wet swale treatment processes and it can only be assumed that the treatment processes are similar to those of a wetland. In the absence of infiltration, biological activity and limited sedimentation are probably important treatment mechanisms. The data available at this time suggest wet swales provide less pollutant removal than dry swales, which might be due to the absence of infiltration.
Siting and Design Considerations
Designers of grassed swales must have site-specific data on topography, depth to seasonal high groundwater, and soil type prior to designing dry or wet swales. Existing topography will establish the general bottom slope of the swale (recommended between one and two percent) and dictate whether check dams will be required. The depth to groundwater is needed to determine if the swale will be of a dry or a wet design. In dry swales the surficial groundwater table should be more than 0.92 m (3 ft) below the proposed invert; wet swales require that the surficial groundwater table is close to the proposed invert. If the depth to the surficial groundwater table and fluctuations in this depth are not considered, it may result in an unacceptable design. Evaluating in situ soil characteristics such as color and structure is helpful in identifying whether excavated soil can be used for the highly permeable soil medium placed below the invert of a dry swale (e.g., a well-drained silty sand).
Dry or wet swales can be designed to treat the first flush of stormwater runoff (frequently taken as the first 12.7 mm [0.5 in] of runoff from the impervious area). In sizing dry or wet swales it is important to define what depth of runoff is associated with the first flush or water quality volume (WQV), as this runoff depth varies from state to state. Swales are configured as on-line facilities; while providing treatment of the WQV for small, frequent storms, swales must still retain the ability to convey high runoff rates from the roadway when high-intensity storms occur. During these larger rainfall events, swales provide marginal treatment of the high flow rates; however, because the flow velocity in the swale is nonerosive, resuspension or transport of accumulated pollutants is minimized.
Pretreatment is not considered crucial to the removal performance of dry/wet swales unless there is sufficient loading of pollutants (e.g., oil and grease) to harm the grassed surface. However, pretreatment (e.g., streetsweeping or forebays) can provide a benefit by reducing and simplifying operation and maintenance of dry/wet swales.
Dry swales provide the majority of treatment by the process of soil infiltration, which filters suspended pollutants and facilitates adsorption of dissolved pollutants. It has been found that the mass removal of pollutants in dry swales is roughly proportional to the mass runoff that infiltrates through the bottom of the channel (Yousef et al., 1985). Even though the residence time in swales can be relatively long (on the order of a day), a review of water monitoring results suggests sedimentation plays a very small role in treatment in dry swales (Claytor and Schueler, 1996).
A dry swale is designed to capture and filter runoff from a water quality rainfall event. In designing a dry swale it is important to first determine the volume of water to be stored. This establishes the basic swale dimensions of width, length, and side slopes. Of equal importance in the design is to select a soil that permits infiltration of the stored stormwater within a reasonable period of time (typically on the order of one day). Infiltration rates for soils are quite variable, even within a single textural class. For example, soils classified as "Loam" may have infiltration rates ranging from 1.5 mm/h to 86 mm/h (0.06 in/h to 3.4 in/h). Computer programs, such as Soil Conservation Service Technical Release 20 Project Formulation Hydrology, can be used to evaluate how effective the storage capacity and infiltration rates of the swales are at attenuating peak stormwater runoff. Additional design procedures and information can be found in Urban Drainage Design Manual Hydraulic Engineering Circular 22 (Brown et al., 1996), Evaluation and Management of Highway Runoff Water Quality (Young et al., 1996), Design of Stormwater Filtering Systems (Claytor and Schueler, 1996), and Highway Runoff Manual (WSDOT, 1995).
In establishing the grassed swale, it is important to check that the swale has sufficient conveyance to drain large rainfall event. Depending on the applicable state or local ordinance this might be as large as the 25-year event. This requirement will establish the minimum size of any culverts and maximum size of any low-flow weirs placed in the swale.
Maintenance Considerations
Maintenance efforts and costs for swales are minimal (Schueler, 1992). Periodic maintenance for dry/wet swales should primarily focus on removing accumulated materials (e.g., sediment and trash or debris). Sediment build-up within the bottom of the swale should be removed when it has accumulated to the point where it occupies approximately 25 percent of the original design volume (Claytor and Schueler, 1996) or when the depth of sediment exceeds 101.6 mm (4 in) (Young et al., 1996). For publicly maintained swales, planners should anticipate removing sediment from 3 to 10 percent of the total swale length for each year of operation (Urbonas et al., 1992).
Maintenance of dry swales includes steps to ensure a vigorous and healthy grass growth. This includes periodic mowing to keep grasses at acceptable levels and minimize the growth of successional vegetation. The frequency of mowing varies with location, but it is recommended that the maximum height of the grass be between 7.62 and 10.2 cm (3 and 4 in) (Claytor and Schueler, 1996). Growth established above the sustained waterline in wet swales must also be maintained; wetland growth will colonize those areas below the waterline. Unfortunately, there is no firm rule for establishing when and where vegetation must be managed so it does not interfere with the basic function of the wet swale. For both dry and wet swales, it is important to avoid the use of herbicides and fertilizers. Particularly in urban areas, the low-lying nature of swales makes them a likely collector of unsightly litter, which must be removed by hand. It is recommended that twice-a-year inspection be performed for litter (Urbonas et al., 1992). One source gives the annual cost of maintaining a grassed swale (in Wisconsin) at between $1.90 and $4.10 (1995 dollars) per linear meter ($0.58 and $1.25 per linear ft) (SWRPC, 1991).
Cost Considerations
Dry and wet swales are considered moderate and low-cost BMPs, respectively. The principal cost difference between the two swale designs arises from the cost of installing highly permeable soils and underdrain systems in a dry swale. The construction cost per hectare served is typically around $3,700 ($1,500 per acre served) based on a nearly flat dry swale with a 3.05 m (10 ft) bottom width, 3:1 side slopes, and a ponding depth of 0.31 m (1 ft). This cost estimate excludes real estate, design, and contingency costs. This unit cost value should be used for conceptual cost estimating only. The cost of a dry/wet swale can also be inferred from the cost of a traditional grass swale, which typically ranges between $16 and $49 per linear meter ($5 and $15 per linear foot) depending on local conditions, swale dimensions, and the degree of internal storage (i.e., check dams) provided (Schueler, 1992).
References
Brown, S.A., S.M. Stein, and J.C. Warner. 1996. Urban Drainage Design Manual Hydraulic Engineering Circular 22. FHWA-SA-96-078. Federal Highway Administration, Office of Technology Applications.
City of Austin. 1995 (draft). Characterization of Stormwater Pollution for the Austin, Texas Area. Environmental Resources Management Division, Environmental and Conservation Services Department, City of Austin, Austin, Texas.
Claytor, R.A., and T.R. Schueler. 1996. Design of Stormwater Filtering Systems. The Center for Watershed Protection, Silver Spring, MD.
Driscoll, E., and P. Mangarella. 1990. Urban Targeting and BMP Selection. Prepared by Woodward-Clyde Consultants, Oakland, CA, for U.S. Environmental Protection Agency, Washington, DC.
Khan, Z., C. Thrush, P. Cohen, L. Kulzer, R. Franklin, D. Field, J. Koon, and R. Horner. 1992. Biofiltration Swale Performance, Recommendations, and Design Considerations. Municipality of Metropolitan Seattle, Water Pollution Control Department, Seattle, WA.
Schueler, T.R., P.A. Kumble, and M.A. Heraty. 1992. A Current Assessment of Urban Best Management Practices - Techniques for Reducing Non-Point Source Pollution in the Coastal Zone. Metropolitan Washington Council of Governments, Department of Environmental Programs, Anacostia Restoration Team, Washington, DC.
SWRPC. 1991. Costs of Urban Nonpoint Source Water Pollution Control Measures. Technical Report No. 31. Southeastern Wisconsin Regional Planning Commission, Waukesha, WI.
Urbonas, B.R., J.T. Doerfer, J. Sorenson, J.T. Wulliman, and T. Fairley. 1992. Urban Storm Drainage Criteria Manual. Vol. 3. Best Management Practices, Stormwater Quality. Urban Drainage and Flood Control District, Denver, CO.
Washington State Department of Transportation (WSDOT). 1995. Highway Runoff Manual. Washington State Department of Transportation.
Young, G.K., S. Stein, P. Cole, T. Kammer, F. Graziano, and F. Bank. 1996. Evaluation and Management of Highway Runoff Water Quality. FHWA-PD-96-032. Federal Highway Administration, Office of Environment and Planning.
Yousef, Y.A., M.P. Wanielista, H.H. Harper. 1985. Removal of Highway Contaminants by Roadside Swales. Transportation Research Record 1017:62-68.
Yu, S.L., S.L. Barnes, and V.W. Gerde. 1993. Testing of Best Management Practices for Controlling Highway Runoff. Virginia Department of Transportation, Report No. FHWA/VA-93-R16, Richmond, VA.
Yu, S.L., R.J. Kaighn, and S.L. Liao. 1994. Testing of Best Management Practices for Controlling Highway Runoff, Phase II. Virginia Department of Transportation, Report No. FHWA/VA-94-R21, Richmond, VA.
Yu, S.L., and R.J. Kaighn. 1995. The Control of Pollution in Highway Runoff Through Biofiltration. Volume II: Testing of Roadside Vegetation. Virginia Department of Transportation, Report No. FHWA/VA-95-R29, Richmond, VA.
|