Stormwater Best Management Practices in an Ultra-Urban Setting: Selection and Monitoring
Monitoring Case Study-Packed Bed Filter BMP Near Lake Beardall, Orlando, Florida
This case study is based on an evaluation of a packed bed filter by Dyer, Riddle, Mills, & Precourt, Inc. (1995).
In response to growing concerns over long-term water quality issues in the Clear Lake drainage basin, the city of Orlando proposed using vegetated rock media filters to treat urban stormwater runoff that drains into the lake. The Clear Lake drainage basin consists of over 777 ha (3 mi2) of highly developed urban area. Because of land constraints, conventional BMPs were considered to be impractical. The Florida Department of Environmental Regulation provided funding for the construction and research of a packed bed filter system. In December 1990, Dyer, Riddle, Mills, & Precourt, Inc. (DRMP) were retained by the city of Orlando to perform the engineering design of the project. Because remedial measures to implement water quality improvements for the entire basin were cost-prohibitive, DRMP prepared a design for a packed bed filter in the vicinity of Lake Beardall to remove pollutants from a 48.9 ha (120.9 ac) basin of highly industrial land use. The system consists of a storage treatment pond used for sedimentation and detention, followed by a packed bed filter system planted with wetland macrophytes for nutrient uptake.
Study Objectives
-
Evaluate the effectiveness of the packed bed system as a whole.
-
Evaluate the effectiveness of each packed bed separately.
-
Estimate the pollutant removal efficiency for three different flow rates.
Design and Operation
The design of the packed bed filter treatment system consists of (1) an off-line storage facility to capture the first flush (first 12.7 mm or first-half inch of a 2-year storm) of stormwater runoff; (2) implementation of diversion weirs to shunt the first flush to the storage facility while allowing the remaining stormwater runoff to bypass the facility; (3) creation of a sedimentation area within the storage facility; (4) creation of 10 packed bed filters consisting of five crushed concrete and five granite media beds, vegetated with native aquatic plants; (5) use of two pumps to supply water to the packed beds from both the storage facility and Clear Lake; (6) control valving to allow for varied water flow rates through the packed beds; and (7) automated flow measurement combined with composite samplers to quantify pollutant removal within the packed beds. Clear Lake is used as a source of continuing flow during dry periods to maintain the planted beds.
To determine the optimum residence time for pollutant removal using different flow rates, separate valves and meters were installed for each filter bed. The packed filter system consists of 10 beds that are individually excavated cells, measuring 24.4 m (80 ft) wide by 9.1 m (30 ft) long by 1.06 m (3.5 ft) deep. Each filter bed is lined with a plastic liner to help eliminate the influence of groundwater and filled with 91.4 cm (36 in) of 3.8 to 7.6 cm (1.5 to 3 in) diameter crushed concrete or granite. Header pipes are installed on the inflow and outflow ends to provide equivalent cross-sectional flow through the filter medium. To ensure that an even water level was maintained in each filter bed because of varying flow rates, outfall weirs, consisting of 10.1 cm (4 in) PVC risers, were installed. An important component of the filter system was the design of a stormwater detention pond. Since the pollutant removal mechanism of a packed bed filter is similar to a trickling filter, the packed beds required a slow, constant flow rate to effectively remove particulate matter. Because stormwater runoff occurs at high flow rates for short periods of time, a detention pond was designed to capture the first-flush runoff, settle out larger particulate matter, and provide a slow, steady flow to the filter beds for treatment. Native vegetation was planted into the rock without soil to enhance the pollutant removal capabilities of the filter. The vegetation consisted of maidencane, giant bulrush, and fireflag, as illustrated in Table 42. The treated stormwater runoff is collected from the controlled outlets into a 300 mm (12 in) PVC collection pipe. The collection pipe transports the effluent through gravity flow to an existing 900 mm (36 in) storm drain outfall point on the drainage canal.
Table 42. Medium type and vegetation for packed bed filter system
Filter |
Media Type |
Vegetation Type |
1 |
Crushed Concrete |
Maidencane |
2 |
Crushed Concrete |
Giant Bulrush |
3 |
Crushed Concrete |
Fireflag |
4 |
Crushed Concrete |
Giant Bulrush & Fireflag |
5 |
Crushed Concrete |
Void of Vegetation |
6 |
Gravel |
Void of Vegetation |
7 |
Gravel |
Giant Bulrush & Fireflag |
8 |
Gravel |
Fireflag |
9 |
Gravel |
Giant Bulrush |
10 |
Gravel |
Maidencane |
Monitoring Program
To determine the pollutant removal efficiency rates of the packed bed filters, automated, flow-proportional composite samplers were installed at the inlet and outlet of the total packed bed filter system. Figure 66 shows a schematic of the packed bed filter sampling stations. To sample the overall inflow to the beds, one sampler was placed at the storage facility pump (see Figure 66, station 1) with the intake tube fitted inside the pump wet well. The flow-proportional sampling was set such that 100 mL were taken every 10 minutes of pump run time, thus producing a composite flow-proportional sample. Another sampler was placed directly downline of the confluence of all individual bed outfalls to provide data on the overall bed system outflow. By determining the time required for a particle of water to flow through the bed system (residence time) and delaying the start of the outflow sampler by the residence time, the entire removal efficiency for the packed bed system can be determined. The range of residence times varied between 6 hours and 24 hours depending on the flow rate. During the monitoring program, three distinct flow rates were used to evaluate removal efficiencies: 454.2 L/min, 227.1 L/min, and 113.5 L/min (120 gal/min, 60 gal/min, and 30 gal/min). The sampling period lasted for nearly four months, at which time 15 simulated storm events were sampled at various flow rates.
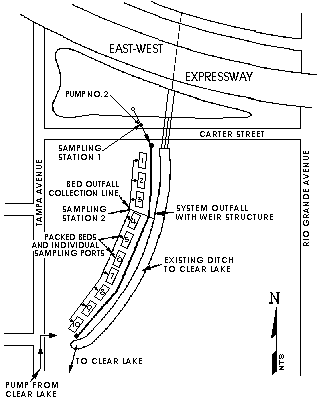
The samples were analyzed for the following constituents: cadmium, chromium, copper, lead, zinc, ammonia, total dissolved solids (TDS), total suspended solids (TSS), total Kjeldahl nitrogen (TKN), total nitrogen (TN), total phosphorus (TP), orthophosphate, nitrate, nitrite, and volatile suspended solids (VSS). Grab samples were taken for the same 15 events from a spigot on the pump 2 (station 1) discharge line and from the system outfall (station 2) and analyzed for fecal coliform (FC) and total organic carbon (TOC). Pollutant loads entering and leaving the entire packed bed system were determined using a mass balance approach. To evaluate the pollutant removal rates of individual packed beds, water quality samples were taken of the inflow and outflow of each bed separately. In an effort to save costs, it was assumed that the inflow to all beds was well mixed; therefore, the composite sampler of station 1 was used to collect a single inflow composite sample representing the inflow concentrations of all beds. Due to time and budget constraints, grab samples were taken at each bed outfall structure. The overall system removal efficiency is given in Table 43. Table 44 gives the overall system removal efficiency for three different flow rates. A discussion of the individual bed performances is provided in the conclusions.
Table 43. Overall system mass balance removal efficiency
Parameter |
Removal Efficiency (%) |
Cadmium |
80 |
Chromium |
38 |
Copper |
21 |
Lead |
73 |
Zinc |
55 |
TN |
63 |
TKN |
62 |
Ammonia |
6 |
Nitrate |
75 |
Nitrite |
9 |
TP |
82 |
Orthophosphate |
14 |
TDS |
8 |
TSS |
81 |
VSS |
80 |
TOC |
38 |
FC |
78 |
Table 44. Overall system removal efficiency (%) for three different flow rates
Parameters |
High Flow Rate |
Mid Flow Rate |
Low Flow Rate |
Cadmium |
71 |
52 |
90 |
Chromium |
27 |
18 |
36 |
Copper |
60 |
26 |
33 |
Lead |
85 |
70 |
77 |
Zinc |
70 |
46 |
60 |
TN |
69 |
60 |
73 |
TKN |
69 |
57 |
73 |
Ammonia |
40 |
10 |
15 |
Nitrate |
-55 |
57 |
73 |
Nitrite |
-5 |
50 |
73 |
TP |
83 |
71 |
80 |
Orthophosphate |
4 |
-1 |
-49 |
TDS |
3 |
18 |
31 |
TSS |
85 |
83 |
88 |
VSS |
80 |
83 |
88 |
TOC |
20 |
15 |
16 |
FC |
74 |
79 |
-46 |
Conclusions
-
The average percent removals in Table 44 for cadmium, chromium, TN, TKN, nitrate, nitrite, TDS, TSS, and VSS seem to indicate that the low flow rate is the most effective in removing these parameters. However, when the need for available storage within the detention pond and the mass of pollutant removed per hour of operating time are factored in, the high rate is the most cost-effective alternative flow rate for all parameters except nitrate, nitrite, and TDS.
-
The concrete media control (bed 5) was consistently better at pollutant removal than the granite media control (bed 6). One possible explanation is that the difference in pH (7.5 in the concrete vs. 6.9 in the granite beds) influenced biological and chemical reactions within the beds. Another explanation is that the different textures of the media affected the amount and type of epilithic algae that was able to grow. Within the concrete media beds, the vegetated beds exhibited no advantage over the control bed (unvegetated bed 5) in the removal of any of the pollutants studied.
-
In contrast to the concrete beds, the vegetated granite media beds provided additional pollutant removal when compared to the unvegetated granite control bed. With the exception of Pb, ammonia, TN, TSS, VSS, and FC, removal efficiencies for the vegetated granite media beds were statistically significant at the 5 percent level of significance.
References
Dyer, Riddle, Mills & Precourt, Inc. 1995. Packed Bed Filter. Final Report, Project No. SP214. Prepared for City of Orlando Stormwater Utility Bureau. Submitted to the Florida Department of Environmental Protection.
|