Stormwater Best Management Practices in an Ultra-Urban Setting: Selection and Monitoring
Stormwater BMPs, both structural and nonstructural, have been used to mitigate the effects of stormwater runoff on receiving water bodies. Structural BMP techniques operate by trapping and detaining runoff so that stormwater constituents settle out or are filtered and trapped by the underlying soil or media. The basic mechanisms for removal of constituents are gravity settling, infiltration of soluble nutrients through the soil profile or filter media, or biological and chemical processes. Structural BMPs might use one or more of these mechanisms to achieve constituent removal from stormwater runoff. Nonstructural BMPs are typically "source control" measures, designed to reduce the level of contaminants and their concentrations in stormwater runoff.
3.1 Overview of BMPs
This chapter presents design guidance to evaluate the different types of BMPs, both structural and nonstructural, and their applicability for a given ultra-urban site. The different BMP technologies have been grouped according to primary treatment mechanisms. The sections are outlined below:
Structural BMPs
3.2 - Infiltration Practices/Bioretention
3.3 - Detention and Retention/Wetland Practices
3.4 - Filtration Practices/Sand Filters
3.5 - Vegetated Swales/Filter Strips
3.6 - Water Quality Inlets
3.7 - Porous Pavements
Nonstructural BMPs
3.8 - Streetsweeping
3.9 - Other nonstructural BMPs (source control)
New and Innovative Practices
3.10 - New and Innovative Practices
Most of the sections contain summary 'fact sheets" that give key information on specific BMP technologies and include carefully collected data on applicability, effectiveness, siting and design considerations, maintenance considerations, and cost considerations. The BMP technologies highlighted in the fact sheets are directly applicable to ultra-urban settings or areas directly connected to roadways.
These factors were used to distinguish BMPs applicable to ultra-urban settings and BMPs for urban areas that are applicable, under certain circumstances, to ultra-urban settings:
-
Limited space available for BMP implementation (less than 0.5 ha [1 ac]).
-
Drainage area imperviousness greater than 50 percent.
-
Property value of land over $215 per square meter ($20 per square foot).
-
Location of BMP in right-of-way (only available space).
-
Existence of build-out conditions at the site (lot-line to lot-line development).
Table 3 provides a brief summary of each of the BMP technologies covered within each section of Chapter 3. The table indicates whether the information has been presented in a fact sheet format or is provided as part of the section, and whether case study evaluations are also presented. Citations are provided within each section for easy reference to detailed design procedures (if applicable), design detail specifications, and studies where technologies have been evaluated.
Table 3. Overview of BMPs
Section |
Treatment Mechanism |
Common Characteristics |
Technologies Addressed/Fact Sheet (FS) or Case Study (CS) |
Structural BMPs |
3.2 - Infiltration Practices/Bioretention |
adsorption, biodegradation, precipitation |
adequate soil media critical |
Infiltration Trenches (FS) (CS)Infiltration Basins (FS)Bioretention (FS) |
3.3 - Detention and Retention/Wetland Practices |
particulate settling and biological filtering (wetlands) |
adequate hydrology and soils required for retention/wetlands |
Detention Ponds (FS) (CS)Wetlands/Shallow Marsh Systems (FS)Detention Tanks and Vaults (FS) |
3.4 - Filtration Practices/Sand Filters |
straining, adsorption, chemical transformation, microbial decomposition |
effective suspended solids removal |
Underground Filters (FS) (CS)Surface Filters (FS)Organic Media Filters (FS) (CS) |
3.5 - Vegetated Swales/Filter Strips |
infiltration, filtration, adsorption |
low cost, easy to install |
Dry and Wet Swales (FS) (CS)Vegetated Filter Strips (FS) (CS) |
3.6 - Water Quality Inlets |
settling |
mainly pretreatment |
Oil-Grit Separators (FS)Catch Basin Inserts (FS)Manufactured Systems (FS) |
3.7 - Porous Pavements |
infiltration |
regular maintenance essential to prevent clogging |
Porous Pavement (FS) |
Nonstructural BMPs |
3.8 - Streetsweeping |
physical removal of surface build-up |
can be implemented as part of a community-wide program |
Street Sweepers (FS) (CS) |
3.9 - Other Nonstructural BMPs |
source control |
|
New and Innovative Practices |
3.10 - New and Innovative Practices |
various |
under development |
Alum Injection Systems, Multi-Chamber Treatment Train (MCTT), Vegetated Rock Filters, Vertical Filter Systems |
Monitoring studies evaluating the effectiveness of various BMP technologies have been collected to provide original information on the effectiveness of the different BMPs. Figure 2 illustrates the location of the various ultra-urban monitoring studies collected during the literature search process. For illustrative purposes only, these BMPs have been grouped into general categories based on their treatment mechanisms. The categories are filtration systems (e.g., sand and organic media filters); infiltration practices (e.g., infiltration trenches and porous pavements); ponds and wetlands; swales and filter strips; and manufactured/pretreatment BMPs. These studies are in-depth reviews of specific BMPs or components of BMPs. Less than 10 percent of the BMP studies included an evaluation of the larger treatment system, where more than one BMP was used in series. Most of the monitoring studies have been concentrated in four areas of the country: Florida; City of Austin, Texas; Washington, D.C. metro area (including Maryland and northern Virginia); and the Puget Sound Region, Washington. Variations in geographic location, climatic conditions, and physical site constraints may have a significant impact on the results of the monitoring evaluations. When reviewing BMP information, it is advisable to consider the location and sites where they were designed and developed.
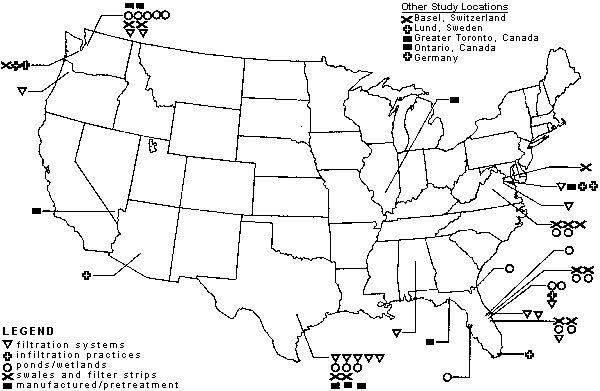
Nonstructural BMPs are presented in Section 3.9. These BMPs are typically "source control" measures and may include land use planning, materials management practices, infrastructure maintenance, and landscaping practices. Nonstructural BMPs may help to minimize the need for more expensive structural controls. They are often used together with structural controls to help improve effectiveness.
Section 3.10 addresses new and innovative practices for the ultra-urban environment.
These technologies have received some preliminary evaluation, but the data are insufficient at this time to provide the type of in-depth discussion that is included in the fact sheets presented in previous sections.
Table 4. Site Considerations
BMP |
Area Typically Served (ha) |
Area Required for BMP7 |
In Situ Soils |
Minimum Head Requirement1 (m) |
Configuration |
Climate a Significant Factor?2 |
Structural BMPs |
Infiltration Trench |
0.8-1.6 |
2-4% |
dependent |
0.9-2.4 |
off-line/on-line |
Yes |
Infiltration Basin |
0.8-8.0 |
2-4% |
dependent |
0.9-1.2 |
off-line |
Yes |
Bioretention |
0.4-20.0 |
4-10% |
independent3 |
0.6-1.2 |
off-line/on-line |
Yes |
Detention Ponds |
0.8 min |
10-20% |
independent |
0.9-1.8 |
on-line |
No |
Wetlands |
0.4 min |
10% |
dependent |
0.3-2.4 |
off-line/on-line |
Yes |
Detention Tanks4 |
0.4-0.8 |
0.5-1% |
independent |
1.5-2.4 |
off-line |
No |
Underground Sand Filters |
0.8-2.0 |
2-3% |
independent |
0.3-2.4 |
off-line |
No |
Surface Sand Filters |
0.8-2.0 |
2-3% |
independent |
1.5-2.4 |
off-line |
Yes |
Organic Media Filters |
0.8-2.0 |
2-3% |
independent |
1.5-2.4 |
off-line |
Yes |
Vegetated Swales |
0.8-1.6 |
10-20% |
dependent |
0.6-1.8 |
on-line |
Yes |
Vegetated Filter Strips |
< 2 |
25%5 |
dependent |
negligible |
on-line |
Yes |
Oil-Grit Separators |
0.4-0.8 |
< 1% |
independent |
0.9-1.8 |
on-line |
No |
Catch Basin Inserts |
< 0.4 |
None |
independent |
0.3-0.6 |
on-line |
None |
Manufactured Systems |
0.4-4 |
None |
independent |
1.2 |
on-line |
None |
Porous Pavements |
0.8-1.6 |
NA |
dependent |
NA |
NA |
Yes |
Nonstructural BMPs |
Streetsweeping |
NA |
NA |
independent |
NA |
NA |
No |
New and Innovative Practices |
Alum Injection |
20-80 |
< 1% |
independent |
0 |
on-line |
No |
MCTT |
0.1-1.0 |
0.5-1.5 |
independent |
1.2-1.8 |
off-line |
Yes |
Biofilters (e.g., StormTreat System) |
0.8-2.0 |
2% |
independent |
1.2-1.8 |
off-line |
Yes |
Vegetated Rock Filters |
0.8-2.0 |
3-5%6 |
independent |
0.6-1.2 |
off-line |
Yes |
NA = Not Applicable or Not Available
Adapted from Claytor and Schueler, 1996; Young et al., 1996; and others.
- Either the depth of water in the typical design or the total drop in water level for flow-through designs.
- Climate issues to consider include prolonged drought and freeze periods.
- When equipped with an underdrain system.
- Based on storage of 12.7 mm (0.5 in) of runoff per acre of imperviousness.
- Minimum recommended for best treatment efficiency.
- Does not include pretreatment/equalization units required for the design.
- Expressed as a percent of the total drainage area, can be modified to accommodate ultra-urban conditions.
|
These technologies, for the most part, appear promising, and ongoing evaluations may provide confirmation of effective performance. For example, the multi-chamber treatment train (MCTT) has been piloted in a full-scale field setting, with promising results. The monitoring results from an installation at a public works garage in Milwaukee, Wisconsin, should provide additional verification of performance.
To help those involved in the BMP selection process, summary tables (Tables 4, 5, and 6) have been developed to assist in comparing and evaluating the various limitations, constituent removal capabilities, and site criteria associated with different BMP technologies. These tables will also be useful in applying the BMP selection process outlined in Chapter 6.
Table 5. Management Considerations
BMP |
Capital Costs |
O&M Costs |
Maintenance |
Training1 |
Effective Life2 |
Structural BMPs |
Infiltration Trench |
Moderate to High |
Moderate |
Sediment/debris removal |
Moderate |
10-15 years |
Infiltration Basin |
Moderate |
Moderate |
Mowing |
Low |
5-10 years before deep tilling required |
Bioretention |
Moderate |
Low |
Mowing/plant replacement |
Low |
5-20 years3 |
Detention Ponds |
Moderate |
Low |
Annual inspection |
Low |
20-50 years |
Wetlands |
Moderate to High |
Moderate |
Annual inspection/plant replacement |
Low |
20-50 years |
Detention Tanks |
Moderate to High |
High |
Frequent cleanout |
Moderate |
50-100 |
Underground Sand Filters |
High |
High |
Annual media removal |
Moderate |
5-20 years |
Surface Sand Filters |
Moderate |
Moderate |
Biannual media cleanout |
Low |
5-20 years |
Organic Media Filters |
High |
High |
Annual media removal |
Low |
5-20 years |
Vegetated Swales |
Low |
Low |
Mowing |
Low |
5-20 years |
Vegetated Filter Strips |
Low |
Low |
Mowing |
Low |
20-50 years |
Oil-Grit Separators |
Moderate |
High |
Frequent cleanout |
Moderate |
50-100 years |
Catch Basin Inserts |
Low |
Moderate to High |
Frequent cleanout |
Low |
10-20 years |
Manufactured Systems |
Moderate |
Moderate |
Periodic cleanout |
Low |
50-100 years |
Porous Pavements |
Low |
Moderate |
Semi-annual vacuum cleaning |
Low |
15-20 years |
Nonstructural BMPs |
Streetsweeping |
Moderate |
NA |
NA |
Low |
4-8 years |
New and Innovative Practices |
Alum Injection |
Moderate |
Moderate |
Periodic chemical resupply |
Low |
5-20 years4 |
MCTT |
High |
High |
Sand filter cleaning & replacement of oil absorbent material |
Low |
5-20 years4 |
Biofilters (e.g., StormTreat System) |
Moderate |
Moderate |
Regular cleanout of accumulated sediment/floatables |
Low |
5-20 years4 |
Vegetated Rock Filters |
High |
High |
Regular inspection and cleanout |
Low |
5-20 years |
NA = Not Applicable or Not Available
Adapted from Young et al., 1996; Claytor and Schueler, 1996; USEPA, 1993; and others.
- In confined space entry is required, then training is placed at a moderate level; otherwise, training requirements are low.
- Assumes regular maintenance, occasional removal of accumulated materials, and removal of any clogged media.
- As a relatively new BMP, the effective life is uncertain. It is reasonable to assume an effective life at least as long as a vegetated swale.
- Estimated based on best professional judgement.
|
Table 6. Pollutant Removal Effectiveness (%)
BMP |
TSS |
TP |
TN |
NO3 |
Metals |
Bacteria |
Oil & Grease |
TPH |
References |
Structural BMPs |
Infiltration Trench1 |
75-99 |
50-75 |
45-70 |
NA |
75-99 |
75-98 |
NA |
75 |
Young et al. (1996) |
Infiltration Basin1 |
75-99 |
50-70 |
45-70 |
NA |
50-90 |
75-98 |
NA |
75 |
Young et al. (1996) |
Bioretention1 |
75 |
50 |
50 |
NA |
75-80 |
NA |
NA |
75 |
Prince George's County (1993) |
Detention Ponds4 |
46-98 |
20-94 |
28-50 |
24-60 |
24-89 |
NA |
NA |
NA |
City of Austin (1990);
City of Austin (1995);
Harper & Herr (1993);
Gain (1996);
Martin & Smoot (1986);Young et al. (1996);
Yu & Benelmouffok (1988);
Yu et al. (1993 & 1994) |
Wetlands |
65 |
25 |
20 |
NA |
35-65 |
NA |
NA |
NA |
USEPA (1993) |
Detention Tanks |
NA |
NA |
NA |
NA |
NA |
NA |
NA |
NA |
|
Underground Sand Filters |
70-90 |
43-70 |
30-50 |
NA |
22-91 |
NA |
NA |
NA |
Bell et al. (1995);
Horner & Horner(1995);
Young et al. (1996) |
Surface Sand Filters |
75-92 |
27-80 |
27-71 |
0-23 |
33-91 |
NA |
NA |
NA |
City of Austin (1990);
Welborn & Veenhuis (1987) |
Organic Media Filters |
90-95 |
49 |
55 |
NA |
48-90 |
90 |
90 |
90 |
Claytor and Schueler (1996);
Stewart (1992);
Stormwater Management (1994) |
Vegetated Swales |
30-90 |
20-85 |
0-50 |
NA |
0-90 |
NA |
75 |
NA |
City of Austin (1995);
Claytor and Schueler (1996);
Kahn et al. (1992);
Yousef et al. (1985);
Yu & Kaighn (1995);
Yu et al. (1993 & 1994) |
Vegetated Filter Strips |
27-70 |
20-40 |
20-40 |
NA |
2-80 |
NA |
NA |
NA |
Yu and Kaighn (1992);
Young et al. (1996) |
Oil-Grit Separators |
20-40 |
< 10 |
< 10 |
NA |
< 10 |
NA |
50-80 |
NA |
Young et al. (1996) |
Catch Basin Inserts |
NA |
NA |
NA |
NA |
NA |
NA |
up to 90 |
NA |
King County (1995) |
Manufactured Systems |
NA |
NA |
NA |
NA |
NA |
NA |
up to 96 |
NA |
Bryant et al. (1995) |
Porous Pavements |
82-95 |
60-71 |
80-85 |
NA |
33-99 |
NA |
NA |
NA |
MWCOG (1983);
Hogland et al. (1987);
Young et al. (1996) |
Nonstructural BMPs |
Streetsweeping2 |
55-93 |
40-74 |
42-77 |
NA |
35-85 |
NA |
NA |
NA |
NVPDC (1992) |
New and Innovative Practices |
Alum Injection |
NA |
89 |
78 |
14 |
NA |
NA |
NA |
NA |
Harper (1990)3 |
MCTT |
83 |
NA |
NA |
14 |
95 |
NA |
NA |
NA |
Pitt (1996) |
Biofilters (e.g., StormTreat System) |
95 |
89 |
NA |
NA |
65-98 |
83 |
NA |
NA |
Allard et al. (1996) |
Vegetated Rock Filters |
95 |
82 |
75 |
NA |
21-80 |
78 |
NA |
NA |
DRMP (1995) |
NA = Not Applicable or Not Available. Removal efficiencies may be based on either mass balance or average concentration calculations. The values may originate from evaluation of multiple events or from long-term monitoring. Ranges are provided wherever possible.
- Based on capture of 12.7 mm (0.5 in) of runoff volume. Effectiveness directly related to volume of captured runoff.
- Typical values; actual performance strongly related to the type of equipment, cleaning frequency, and number of passes.
- Study examined improvement in water quality within the lake receiving alum-treated stormwater runoff.
- Included are results for three different types of ponds: extended detention wet pond, wet pond, and extended detention dry pond.
|
References
Allard, L.A., W. Graham, W. Platz, R. Carr, J. Wheeler. 1996. The StormTreat System Used as a Storm Water Best Management Practice. In Proceedings of Watershed `96.
Bell, W., L. Stokes, L.J. Gavan, T.N. Nguyen. 1995. Assessment of the Pollutant Removal Efficiencies of Delaware Sand Filter BMPs. City of Alexandria, Department of Transportation and Environmental Services, Alexandria, VA.
Bryant, G., F. Misa, D.G. Weatherbe, and W. Snodgrass. 1995. Field Monitoring of Stormceptor Performance, Case Study of Stormceptors in the Greater Toronto Area.
City of Austin. 1990. Removal Efficiencies of Stormwater Control Structures. Environmental Resources Management Division, Environmental and Conservation Services Department, City of Austin, Austin, TX.
City of Austin. 1995 (draft). Characterization of Stormwater Pollution for the Austin, Texas Area. Environmental Resources Management Division, Environmental and Conservation Services Department, City of Austin, Austin, TX.
Claytor, R.A., and T.R. Schueler. 1996. Design of Stormwater Filtering Systems. The Center for Watershed Protection, Silver Spring, MD.
Dyer, Riddle, Mills, & Precourt, Inc. 1995. Packed Bed Filter. Final Report, Project No. SP214. Prepared for City of Orlando Stormwater Utility Bureau. Submitted to Florida Department of Environmental Protection.
Gain, S.W. 1996. The Effects of Flow-Path Modifications on Urban Water-Quality Constituent Retention in Urban Stormwater Detention Pond and Wetland System, Orlando, Florida. Florida Department of Transportation, Orlando, FL.
Harper, H.H. 1990. Long-term Performance Evaluation of the Alum Stormwater Treatment Systems at Lake Ella, Florida. Final Report for FDER Project WM339, submitted to Florida Department of Environmental Regulation.
Harper, H.H., and J.L. Herr. 1993. Treatment Efficiencies of Detention with Filtration Systems. Environmental Research and Design, Inc., Orlando, FL.
Hogland, W., J. Niemczynowice, and T. Wahlan. 1987. The Unit Superstructure during the Construction Period. The Science of the Total Environment (59):411-424.
Horner, R.R., and C.R. Horner. 1995. Design, Construction, and Evaluation of a Sand Filter Stormwater Treatment System, Part II, Performance Monitoring. Report to Alaska Marine Lines, Seattle, WA.
Khan, Z., C. Thrush, P. Cohen, L. Kulzer, R. Franklin, D. Field, J. Koon, and R. Horner. 1992. Biofiltration Swale Performance, Recommendations, and Design Considerations. Municipality of Metropolitan Seattle, Water Pollution Control Department, Seattle, WA.
King County Surface Water Management Division, King County Department of Metropolitan Services, Snohomish County Surface Water Management Division, Seattle Drainage and Wastewater Utility, and Port of Seattle. 1995. Evaluation of Commercially-Available Catch Basin Inserts for the Treatment of Stormwater Runoff from Developed Sites. Interagency Catch Basin Insert Committee.
Martin, E.H., and J.L. Smoot. 1986. Constituent-Load Changes in Urban Stormwater Runoff Routed Through a Detention Pond - Wetlands Systems in Central Florida. U.S. Geological Survey Water Resources Investigations Report 85-4310. Tallahassee, FL.
Metropolitan Washington Council of Governments (MWCOG). 1983. Urban Runoff in the Washington Metropolitan Area: Final Report, Urban Runoff Project, EPA Nationwide Urban Runoff Program. Metropolitan Washington Council of Governments, Washington, DC.
NVPDC. 1992. Northern Virginia BMP Handbook: A Guide to Planning and Designing Best Management Practices in Northern Virginia. Prepared by the Northern Virginia Planning District Commission and Engineers and Surveyors Institute.
Pitt, R.E. 1996. The Control of Toxicants at Critical Source Areas. In Proceedings of Effects of Watershed Development and Management on Aquatic Ecosystems Symposium presented by the Engineering Foundation, Snowbird, UT, Aug. 4-9, 1996.
Prince George's County. 1993. Design Manual for Use of Bioretention in Stormwater Management. Department of Environmental Resources, Prince George's County, Landover, MD.
Stewart, W.S. 1992. Compost Storm Water Treatment System. W&H Pacific Consultants, Portland, OR. Final Report.
Stormwater Management. 1994. Three Year Performance Summary of Stormwater Pollutant and Treatment - 185th Avenue, Hillsboro, Oregon. Technical Memorandum. Stormwater Management, Portland, OR
USEPA. 1993. Guidance Specifying Management Measures for Sources of Nonpoint Pollution in Coastal Waters. EPA-840-B-92-002. U.S. Environmental Protection Agency (USEPA), Office of Water, Washington, DC.
Welborn, C., and J. Veenhuis. 1987. Effects of Runoff Control on the Quality and Quantity of Urban Runoff in Two Locations in Austin, TX. USGS Water Resources Investigations Report 87-4004.
Young, G.K., S. Stein, P. Cole, T. Kammer, F. Graziano, and F. Bank. 1996. Evaluation and Management of Highway Runoff Water Quality. FHWA-PD-96-032, Federal Highway Administration.
Yousef, Y.A., M.P. Wanielista, and H.H. Harper. 1985. Removal of Highway Contaminants by Roadside Swales. Transportation Research Record 1017:62-68.
Yu, S.L., and D.E. Benelmouffok. 1988. Field Testing of Selected Urban BMPs in Critical Water Issues and Computer Applications. In Proceedings of the 15th Annual Water Resources Conference. American Society of Civil Engineers, New York, NY.
Yu, S.L., S.L. Barnes, and V.W. Gerde. 1993. Testing of Best Management Practices for Controlling Highway Runoff. Virginia Department of Transportation, Report No. FHWA/VA-93-R16, Richmond, VA.
Yu, S.L., and R.J. Kaighn. 1992. VDOT Manual of Practice for Planning Stormwater Management. Federal Highway Administration, FHWA/VA-92-R13, Virginia Department of Transportation, Virginia Transportation Research Council, Charlottesville, VA.
Yu, S.L., and R.J. Kaighn. 1995. The Control of Pollution in Highway Runoff Through Biofiltration. Volume II: Testing of Roadside Vegetation. Virginia Department of Transportation, Report No. FHWA/VA-95-R29, Richmond, VA.
Yu, S.L., R.J. Kaighn, and S.L. Liao. 1994. Testing of Best Management Practices for Controlling Highway Runoff, Phase II. Virginia Department of Transportation, Report No. FHWA/VA-94-R21, Richmond, VA.
3.2.1 Description and Purpose
Bioretention areas, infiltration basins, and infiltration trenches can be classified as infiltration practices. Infiltration basins and trenches mainly use the interaction of the chemical, physical, and biological processes between soils and water to filter out sediments and constituents from stormwater. As an added dimension, bioretention areas use the interaction of plants to enhance the treatment process. Constituents are first absorbed, filtered and transformed by the soil and then taken up by the plant roots. In the ultra-urban environment facilities that incorporate infiltration may be off-line or on-line. Off-line facilities usually capture and treat the "first flush" of stormwater, which contains the highest concentration of pollutants. Larger storm events are diverted around the off-line infiltration facility, perhaps into another management system such as an extended detention pond. On-line facilities pass all of the stormwater through the system.
If the in situ soils in an ultra-urban area possess medium to high infiltration rates, infiltration practices can be extremely effective ultra-urban BMPs for the removal of pollutants, such as metals and nutrients from the stormwater. Bioretention areas and infiltration trenches can be used to serve small drainage areas, or they can be installed in larger drainage areas, such as roadway interchanges, to manage large quantities of runoff.
One of the issues encountered with using infiltration practices as ultra-urban BMPs is the potential for groundwater contamination. Recent research in Europe on a series of infiltration systems that had been installed for 12 to 45 years indicated roadway runoff can influence the concentration of heavy metals and polyaromatic hydrocarbons (PAHs) in the underlying soils. However, this study also found that pollution concentrations decline with depth in the soil column, reaching background levels at soil depths less than 1.5 m (4.9 ft). Furthermore, the pollutants captured in the soil tended not to repartition back into infiltrating stormwater (Mikkelsen et al., 1996). An earlier evaluation of infiltrating stormwater in sandy soil in Florida seems to confirm the capture of certain heavy metals, particularly lead and zinc (McKenzie, 1988). However, where systems infiltrate directly into highly permeable soil (gravelly) layers without passing through fine-grain soil layers, stormwater pollutants appear to have a greater influence on surficial groundwater aquifers (Adolfson Associates, 1995).
3.2.2 Design Alternatives
For each of the technologies discussed in the previous section, testing of in situ soils must be done to determine if the infiltration rates of the soils are acceptable. It is important that infiltration trenches and basins have sufficient clearance above the seasonal high groundwater level and any impermeable soil layers (e.g., fragipans). Captured stormwater must be able to infiltrate through all soil layers so that the system will drain before the next storm event. Bioretention area designs are also affected by groundwater levels and in situ soils. However, if there is insufficient clearance above the groundwater or an impermeable soil layer, underdrains can be installed to drain the area.
In an ultra-urban setting, bioretention areas are ideally suited to address water quality issues and can be used for a range of drainage areas. A bioretention area consists of a depressed planted area that retains and infiltrates stormwater through a carefully engineered soil that is typically 1.22 m (4 ft) deep. The depressed area is heavily planted with trees, shrubs, and groundcovers that can withstand urban conditions (e.g., heat, salt, drought conditions) and tolerate frequent inundation. The planted vegetation provides a number of benefits, including a habitat area for urban wildlife. Pretreatment of stormwater flowing into bioretention areas is recommended to remove large debris, trash, and sand.
Infiltration trenches are used primarily to provide water quality treatment for small drainage areas. They can be sited in roadway medians or adjacent to rights-of-way. The trench is filled with large stones, 25 to 75 mm (1 to 3 in) in diameter. The stormwater is stored in the pore space between the stones and then slowly infiltrates into the soils below. The depth of the stones is typically from 1.2 to 2.4 m (4 to 8 ft). Stormwater enters the trench either by sheet flow across the top of the stones or from the outfall of a storm drain system. It is recommended that some type of pretreatment of stormwater, such as a sand filter or oil grit separator, be used to remove sediments from the system. Sediments can reduce the storage capacity and clog the pore spaces of the subsoils, which reduces the infiltration rate into the trench.
Infiltration basins are typically used to manage large volumes of stormwater. Due to the amount of space required, particularly for excavation, infiltration basins typically are limited to roadway interchanges and large residual parcels of land in the right-of-way and may not be suitable for ultra-urban areas. It is recommended that some type of pretreatment, such as buffer strips or a dry, grassed swale, be used to remove sediments from the system.
References
Adolfson Associates, Inc; Brown and Caldwell. 1995. Pilot Evaluation: Subsurface Stormwater Disposal Facilities. Prepared for Tacoma-Pierce County Health Department. Final Report.
McKenzie, D.J., and G.A. Irwin. 1988. Effects of Two Stormwater Management Methods on the Water Quality of Water in the Upper Biscayne Aquifer at Two Commercial Areas in Dade County, Florida. U.S. Geological Survey Water Resources Investigations Report 88-4069, Tallahassee, FL.
Mikkelsen, P.S., M. Hafliger, M. Ochs, J.C. Tjell, P. Jacobsen, and M. Boller. 1996. Experimental Assessment of Soil and Groundwater Contamination From Two Old Infiltration Systems for Road Runoff in Switzerland. The Science of the Total Environment, 189/190:341-347.
|